The Moon's Highs and Lows
Download a paper from the Open Science Framework on sub-topic 'The Tallest' (DOI: 10.31219/osf.io/agpr4).
From Earth we always see one side of the Moon (the nearside) and never the other (the farside) because our satellite takes the same amount of time to spin on its axis as it does to orbit us. It wasn't until 1959 that we first got an inkling of how radically different the farside is when the Soviet probe Luna 3 sent back a few fuzzy pictures of it. Whereas much of the nearside is flat and smooth, the farside is rugged and heavily cratered up north, and a giant splotch occupies most of the south. It took a few more years and better photos for the existence and extent of this mega-dent to be confirmed. But thanks to newer spacecraft like the Lunar Reconnaissance Orbiter (LRO), we've now mapped the entire Moon in exquisite detail. So let's go exploring our rocky companion's highest, lowest and tallest spots.
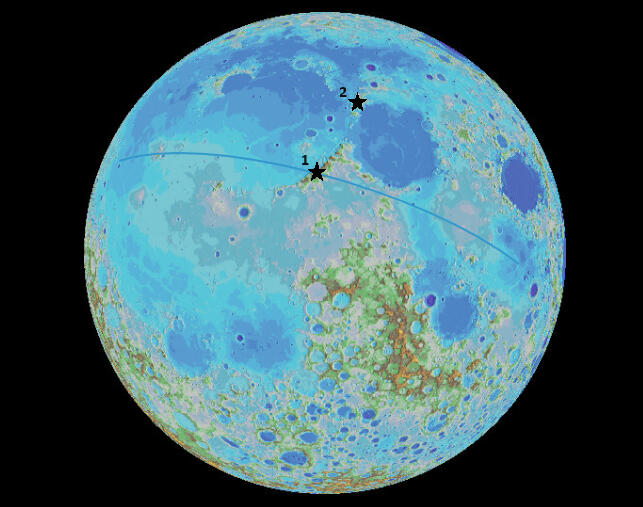
Fig 1: Nearside (LROC Quickmap)
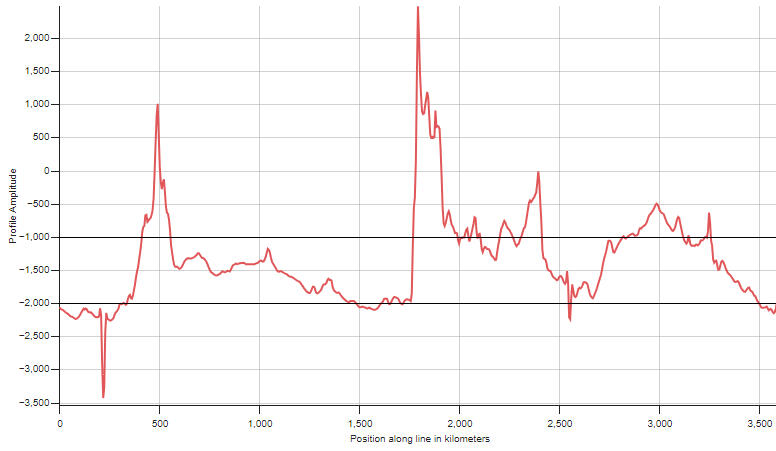
Fig 2: Nearside height profile (LROC Quickmap)
Let's kickoff with the nearside. Fig 1 is what you see when you look up at the full Moon (without the false colour coding of terrain height, that is). Fig 2 is a profile of the terrain height along the transect in Fig 1. Though almost all the landmass along the transect is higher than -2 km (mare level), two-thirds of it is lower than -1 km.
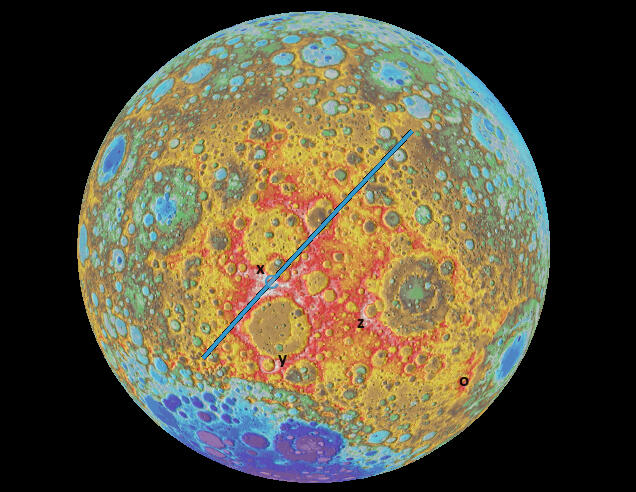
Fig 3: Farside highlands (LROC Quickmap)
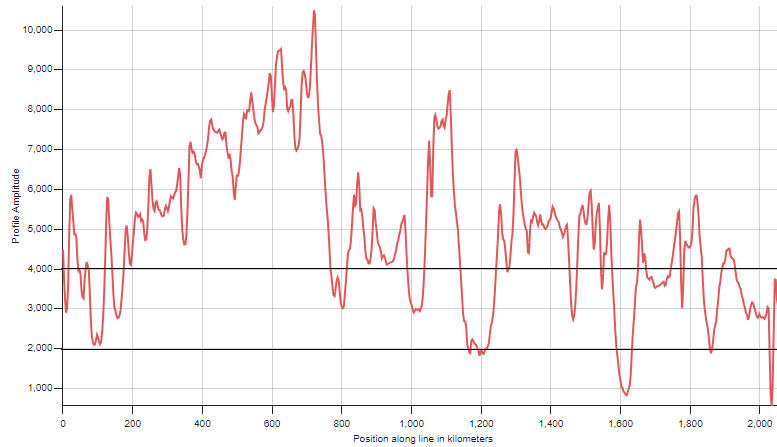
Fig 4: Farside highlands - height profile (LROC Quickmap)
Over on the other side, Fig 3 displays the full expanse of the rugged farside highlands. Fig 4 is a profile of terrain height along the transect in Fig 3. In wild contrast to the lunar nearside, two-thirds of the land mass along the transect is higher than 4 km, and almost all of it is higher than 2 km.
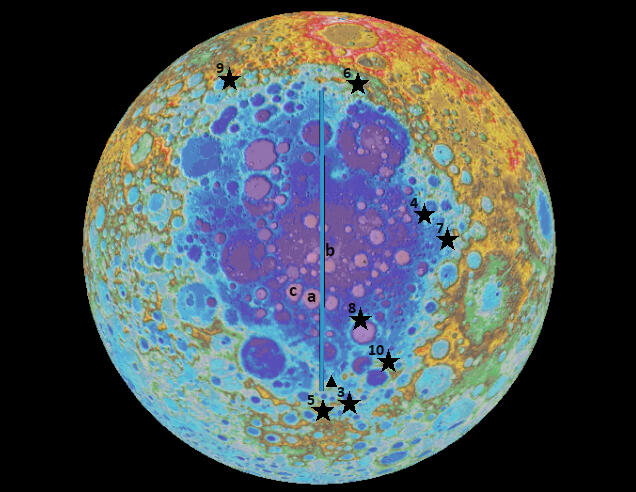
Fig 5: South Pole-Aitken basin (LROC Quickmap)
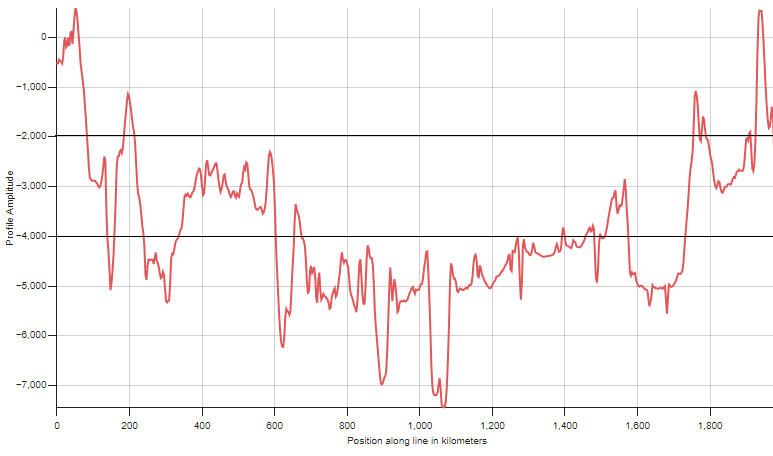
Fig 6: South Pole-Aitken basin - height profile (LROC Quickmap)
Fig 5 is the farside again, but now rotated to clearly show the extent of one of the oldest, largest and deepest craters in the solar system - the South Pole-Aitken (SPA) basin - so named because it extends from the lunar South Pole (marked by a black triangle) to Aitken crater (star '9'). Fig 6 is a profile of terrain height along the transect in Fig 5. This time nearly two-thirds of the landmass along the transect is lower than -4 km, and almost all of it is lower than -2 km.We'll discuss all the other stars and labels in Figs 1, 3 and 5 in subsequent sections. But here's the key for all the colour-coded relief in those figures. Elevation ranges are in meters. The datum, or 0 height reference is taken to be the Moon's mean radius of 1737.4 km - it's the lunar equivalent of sea-level:
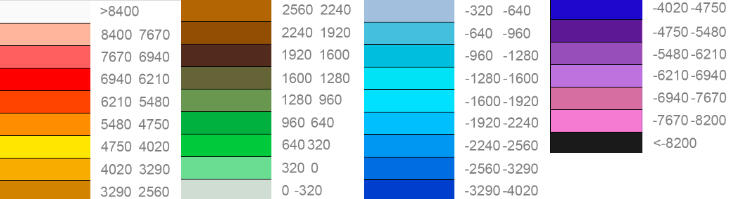
The Highest
Where would you expect to find the highest point on the Moon? Yep, in the farside highlands. The transect in Fig 3 actually passes through the highest point - Selenean Summit (marked by a blue circle) - and its elevation of 10.785 km makes it almost 2 km higher than Mount Everest, the highest point on Earth. There's actually at least a couple of thousand square kilometers of terrain higher than Everest in the white-coded region in which Selenean Summit is located. Besides this area, there's a handful of other particularly high spots in the highlands, listed in Table 1. More about these locations a little later.
Location | Reference | Co-ordinates | Elevation |
---|---|---|---|
Selenean Summit | Fig3 (marked 'x') | [Lat 5.411, Lon 201.367] | 10.785 km |
Orientale basin rim | Fig3 (marked 'o') | [Lat -21.044 Lon 247.673] | 9.460 km |
Vavilov crater rim | Fig3 (marked 'z') | [Lat -2.121 Lon 220.349] | 9.334 km |
Doppler crater rim | Fig3 (marked 'y') | [Lat -12.848 Lon 201.817] | 9.223 km |
Table 1: Moon's highest regions (LROC Quickmap)
The Lowest
Unsurprisingly, the Moon's lowest point is in the SPA basin on the farside. Informally called 'Point Trieste', it sits in a crater within a crater within a crater: in a part of the SPA basin already at -4 km elevation, a projectile gouged out the 140 km Antoniadi crater, the floor of which dips another 3 km, bringing us to about -7 km elevation. Continuing along Antoniadi's floor we encounter a 13 km crater that has carved a further 2 km into the surface, dropping us to over 9 km below the lunar datum. This location is marked 'a' in Figs 5 and 7, and Fig 8 shows the height profile across it.
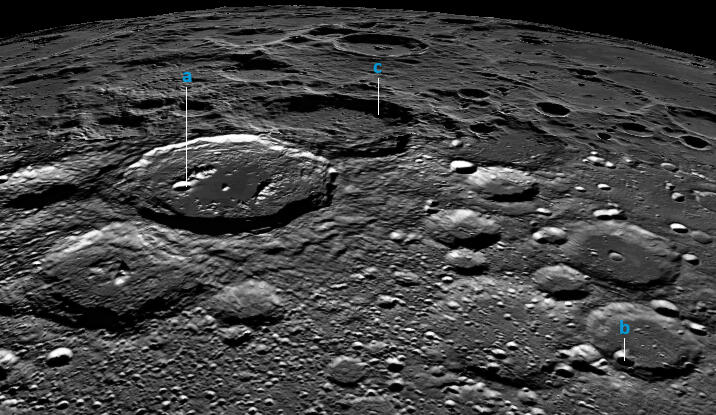
Fig 7: Moon's lowest points - 3D terrain view (LROC Quickmap)
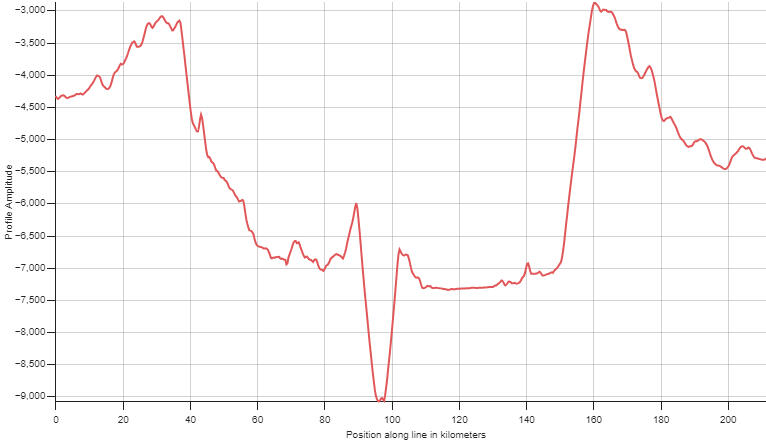
Fig 8: Antoniadi crater - height profile (LROC Quickmap)
There's a few other such 'nested crater' sets in this part of the Moon deeper than 8.5 km, like Chaffee, Chawla, Lemaitre and Minkowski. But the three deepest are listed in Table 2.
Location | Reference | Co-ordinates | Elevation |
---|---|---|---|
'Point Trieste' inside 13 km crater on Atoniadi crater floor in SPA basin | Figs 5 and 7 (marked 'a'), Fig 8 | [Lat -70.36, Lon 187.508] | -9.129 km |
Inside 12 km crater on Bellingshausen crater floor in SPA basin | Figs 5 and 7 (marked 'b'), Fig 9 | [Lat -61.022 Lon 196.194] | -8.888 km |
Inside 7 km crater on Minnaert crater floor in SPA basin | Figs 5 and 7 (marked 'c'), Fig 10 | [Lat -66.408 Lon 176.116] | -8.872 km |
Table 2: Moon's lowest points (LROC Quickmap)
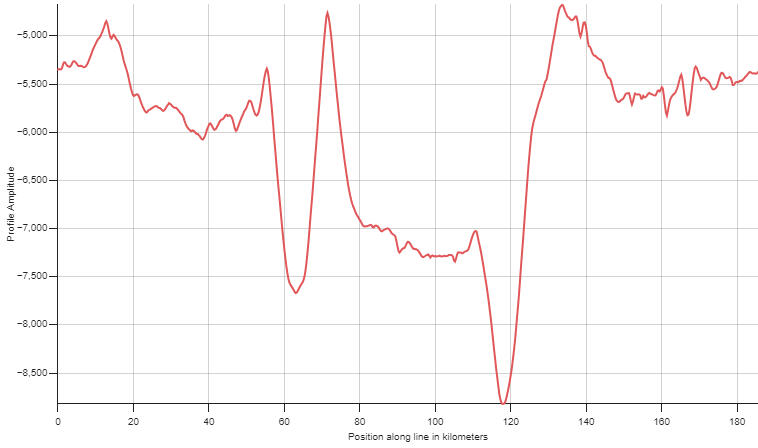
Fig 9: Bellinghausen crater - height profile (LROC Quickmap)
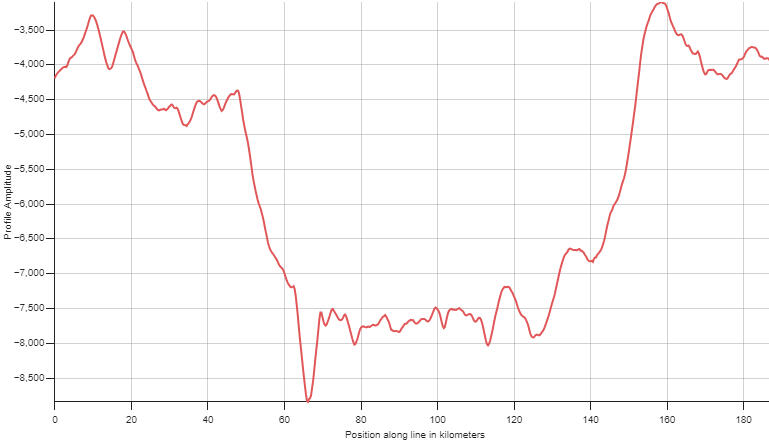
Fig 10: Minnaert crater - height profile (LROC Quickmap)
Bellinghausen is less than half the diameter of Antoniadi and shallower, but has formed in a slightly deeper part of the SPA basin and so its floor is at a similar elevation as Antoniadi's. The small crater on Bellinghausen's floor (let's call it a 'sc') is also about the same size as Antoniadi's, so the Bellinghausen set's overall depth comes to within a whisker of Antonialdi's. I feel a bit sorry for Minnaert though. Its floor is lower than Antoniadi's, but its sc is piddly small, and that's robbed it of the additional depth that Antoniadi and Bellinghausen have gained from theirs. Had Minnaert's sc been similar to that of the others, its set would have come out tops for depth.It's interesting to note that the difference in elevation between the highest and lowest points on the Moon is pretty much the same as on Earth. See Table 3 for a comparison. This is remarkable when we're talking about two worlds of very different size (and so surface gravity), with radically different geological histories - Earth's topography has been primarily shaped by plate tectonics, water and atmosphere, and the Moon's by impacts.To put this coincidence into perspective, consider that the difference in topographical extremes on Mercury is 10 km less than that of Earth and the Moon, while on Mars, it is 10 km greater.
Planet | Highest Point | Lowest Point | Elevation Difference |
---|---|---|---|
Earth | 8.848 km (Mount Everest) | -10.984 km (Challenger Deep) | 19.8 km |
Moon | 10.785 km (Selenean Summit) | -9.129 km (Point Trieste) | 19.9 km |
Table 3: Earth and Moon's highest and lowest elevations (LROC Quickmap)
The Tallest
Where to hunt for the Moon's tallest mountains? To help answer this, let's first decide what a mountain is (yes you'd probably know one if you saw one, but still...), then how Moon mountains are made. According to Britannica:Mountain - landform that rises prominently above its surroundings, generally exhibiting steep slopes, a relatively confined summit area, and considerable local relief.So elevation alone isn't enough for a feature to qualify as a mountain. As peakjut says:A tiny mound on the Tibetan plateau could have a higher elevation than a massive mountain that rises from close to sea level, such as Mount Rainier.But even a 'peak' high above sea level (or the lunar datum as the case may be), that's not on a plateau, is still not a mountain if the terrain slopes up only say, 1° from all sides to reach that point, since there's nothing about such a formation that 'stands out' - there isn't considerable local relief - basically, it isn't prominent. A couple of ways to measure prominence are:a) How far you need to descend from a peak before you can start climbing a higher peak
b) The height of a peak above the lowest contour line fully encircling itIn either case - how far to descend or the lowest closed contour - can be taken as the base of the rise. Consider Selenean Summit - marked by the small blue circle in Fig 11. A contour which encloses it (and skirts around the rim of Englehardt crater) is at an elevation of 9 km, highlighted by the black dots, and Fig 12 shows the profile of a transect across it. The graph reveals that this terrain slopes quite gently - rising 5.5° from 9 km to the summit from the left margin, and 3.5° from the right.
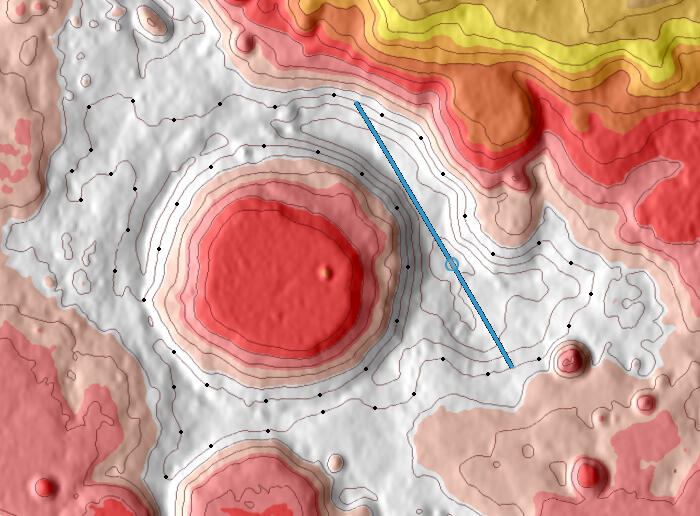
Fig 11: Selenean summit region - elevation contour (LROC Quickmap)
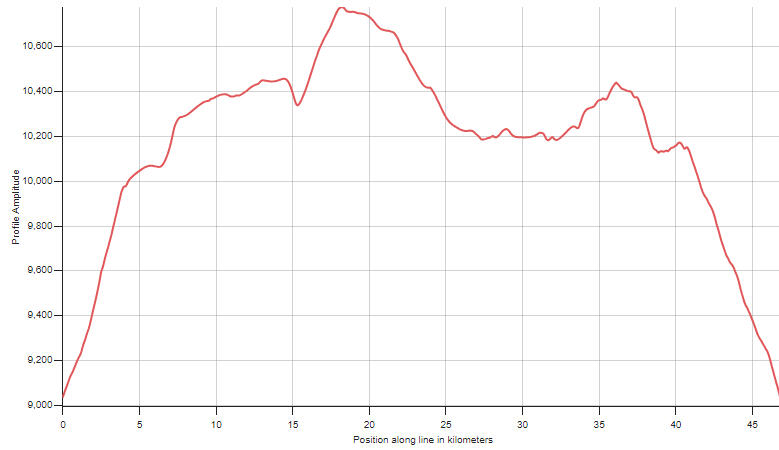
Fig 12: Selenean Summit - height profile (LROC Quickmap)
Selenean Summit's lack of prominence is also clear from the oblique 3D view of the region in Fig 13. Which means neither the Moon's highest or next highest points in its vicinity, are what we'd call mountains.
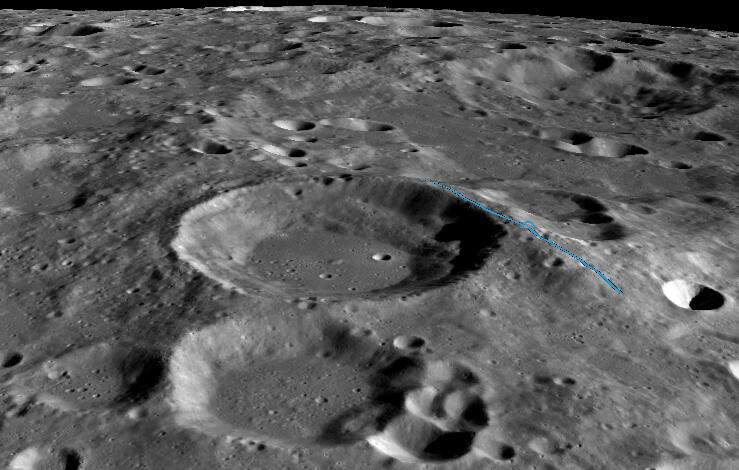
Fig 13: Selenean Summit - 3D terrain view (LROC Quickmap)
Of course the Moon does have mountains, but unlike those on Earth which formed over hundreds of thousands of years from colliding tectonic plates, the lunar ones sprung up virtually instantly as meteoroids slammed into its surface at hypersonic speeds. Celestial impacts create not only a depression many times the size of the projectile, but also regions of raised terrain - including ridges - which arise both from uplift of the bedrock from rebound, and excavated ejecta landing on top. Where these ridges appear, how they look and how tall they are, varies with the type of crater, which depends on the size of the impact:Craters 20 km or smaller are typically bowl shaped with upturned rims (the elevated bit in such craters). Craters larger than this feature a central peak or cluster of peaks, in addition to the raised rim. Normally the height of these peak(s) is lower than that of the rim, but there are exceptions, like the farside crater Icarus. When the crater diameter exceeds about 200 km, the central uplift breaks up - it collapses downward and outward producing a ring of peaks. This ring typically appears at about half the rim diameter. Above 600 km in diameter, multiring basins with three or more concentric peak rings are produced. Fig 14 shows examples of each.
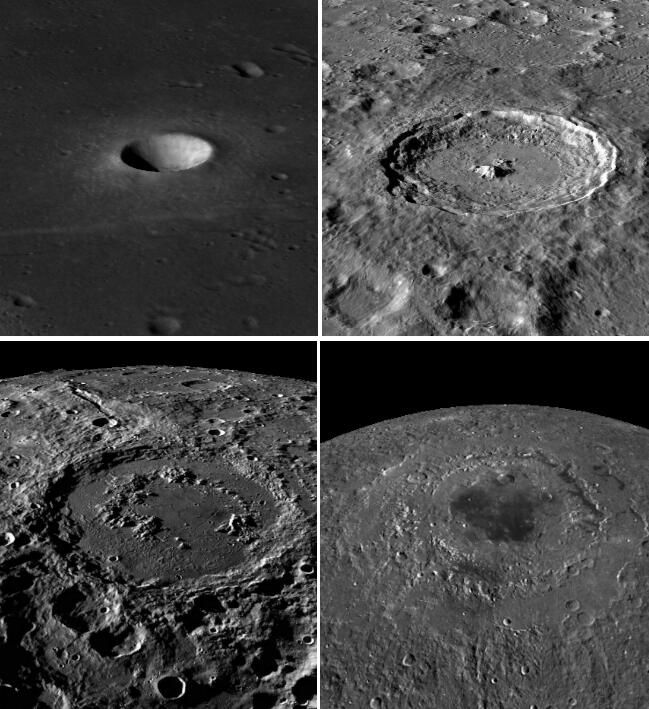
Fig 14: Crater peak structures with increasing crater size - 3D terrain view (LROC Quickmap)
Fig 14 Top left: Moltke. A 6 km 'simple' crater with rim 300m higher than the flat mare in which the crater formed
Fig 14 Top right: Tycho. An 85 km 'complex' crater with rim on average 1 km higher than surrounding terrain and central peak 2.5 km high
Fig 14 Bottom left: Schrödinger. A 340 km 'peak ring' crater with rim on average 2 km higher than surrounding terrain and peaks up to 2.6 km high in the peak ring
Fig 14 Bottom right: Oriantale. A 960 km 'multi-ring' basin with peak heights in 4-5 km range in both the outer (third) and second rings
What these examples makes clear is that the bigger the crater, the deeper it is and the taller its peaks. Which means our best bet for locating the Moon's very tallest peaks is to examine the rings, or what remains of them, of its largest basins. And when we do, the 1200 km Imbrium basin on the nearside, and especially the 2200 km SPA basin on the farside, deliver the goods!As an aside, the excavation of these big basins also results in a massive amount of material being ejected and dispersed far beyond their boundaries. So there's little doubt that ejecta from the ginormous explosion that formed the SPA basin played a role in shaping the elevation of the farside highlands. In fact, it's even been proposed that much of these highlands consists of SPA ejecta. The highest of the high spots have likely resulted from 'crater stacking'. Just as 'crater nesting' (a crater formed in the floor of an existing crater) makes it deeper, so too the rim crest and ejecta blanket of a crater formed on terrain already uplifted by an existing crater, adds to the overall height of the surrounds. For example:The 430 km Korolev crater formed in a region of the thickest crust and further elevated the terrain around its rim, including the area immediately to its north marked 'x' in Fig 3. Engelhardt crater later formed in this area, uplifting its neighbourhood even more - Selenean Summit is located on its eastern rim crest. Similarly 100 km Doppler crater formed on the southern rim of Korolev, contributing to the notably high elevation marked 'y' in Fig 3, around the eastern part of Doppler's rim.Same story with area 'z' in Fig 3: The 580 km Herzsprung basin to the west of Korolev formed in an existing high region thanks to uplift from the SPA basin. Hertzsprung is a basin of intermediate size - transitional between two-ring ones such as Schrödinger and multi-ring ones like Orientale. Again its outer ring of mountains and ejecta blanket have given the area an additional boost. Finally 100 km Vavilov formed on the south-western section of Herzsprung's outer rim, resulting in the especially high elevation around the south-western part of Vavilov's rim.Speaking of Oriantale, the Moon's second highest spot after the Selenean Summit region, marked 'o' in Fig 3, appears to be associated with that famed 'bullseye' basin. In fact, it seems to be a particularly elevated part of Oriantale's outer ring. It may have resulted from an oblique basin-forming impact. Or the exceptional height here may have been influenced by an older, degraded basin that shaped the outcome of the Orientale impact. Unlike regions 'x', 'y' and 'z' though, 'o' is a proper mountain range. For the purposes of this discussion, let's just call the highest peak in this range Oα (for 'Orientale Alpha').
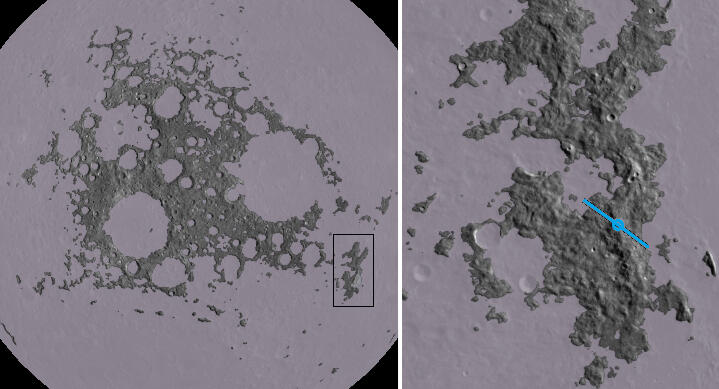
Fig 15: Farside highlands terrain higher than 5 km (LROC Quickmap)
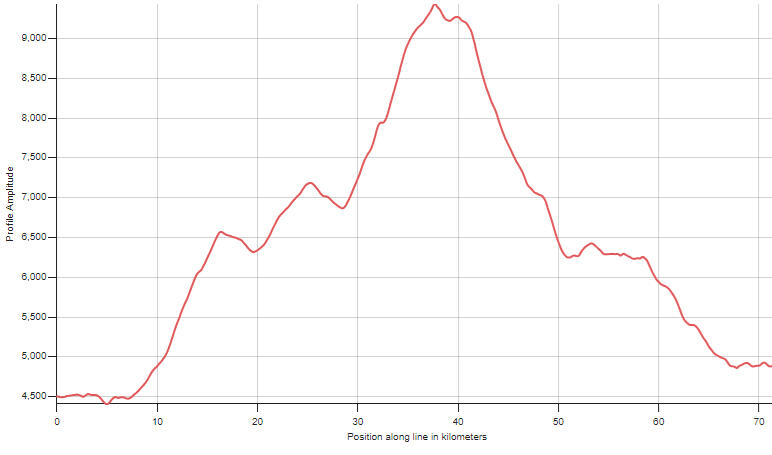
Fig 16: Orientale Alpha* - height profile (LROC Quickmap)
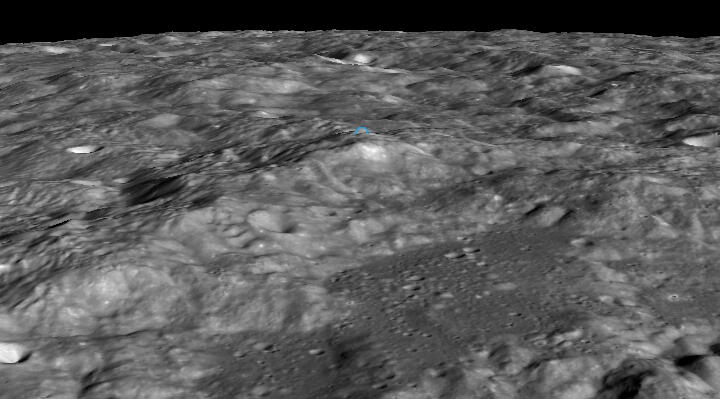
Fig 17: Orientale Alpha* - 3D terrain view (LROC Quickmap)
In Fig 15 left panel shows the terrain of the farside highlands masked to 5 km and higher. This is close to the lowest elevation that allows Oα's mountain range (near the right margin of the image) to remain 'detached' from the surrounding terrain, and so can be taken as its base. Note that this criteria gives a very conservative elevation for the base, compared with say, defining the base elevation to be the lowest point where the mountain's flank 'flattens out'.Per the height profile in Fig 16 along the transect in Fig 15 right panel, the slopes to Oα's summit rise at about 17°.Of all lunar features, Oα's vital stats are closest to that of Mount Everest: Oα's summit's elevation of 9.46 km is a little higher than Mount Everest's at 8.84 km, and Oα's base is at a similar elevation to Mount Everest's on the Tibetan plateau. So the base-to-peak height of both mountains is between 3.5 and 4.5 km. But on this measure, just as Mount Everest isn't among the tallest mountains on Earth, Oα isn't among the tallest on the Moon. These are some of the Moon's tallest mountains:
Name | Peak co-ordinates | Peak Elevation | Base to Peak | Reference |
---|---|---|---|---|
Mons Huygens | [Lat 19.526, Lon 357.096] | 3.274 km | 5.3 km | Fig1 (star '1') |
Drygalski Calipers* | [Lat -76.929, Lon 260.074] | 5.526 km | 5.73 km | Fig5 (star '10') |
Mons Elbruz* | [Lat 39.094, Lon 9.458] | 3.625 km | 5.64 km | Fig1 (star '2') |
Mons Cabeus | [Lat -83.608, Lon 325.971] | 5.689 km | 5.84 km | Fig5 (star '3') |
Mons Twin Peaks* | [Lat -48.195, Lon 227.468] | 4.785 km | 5.78 km | Fig5 (star '4') |
Mons Mouton | [Lat -84.673 , Lon 39.475] | 7.026 km | 6.03 km | Fig5 (star '5') |
Montes Plummer* | [Lat -22.726 , Lon 204.132] | 8.564 km | 6.91 km | Fig5 (star '6') |
Southern Farside Mountain* | [Lat -50.126, Lon 236.785] | 6.739 km | 7.14 km | Fig5 (star '7') |
Table 4: Moon's tallest mountains (LROC Quickmap)
The results in Table 4 are courtesy of the versatile lunar visualization tool LROC Quickmap. Available since 2011, it offers a detailed, interactive topographic representation of the Moon, constructed from high-resolution images and laser-based altitude measurements gathered by the LRO. The upcoming height profile graphs, contour maps and 3D terrain perspectives for each of these entries, have also been generated with LROC Quickmap.The peak elevations are accurate to within 20 meters. For the first and third entries in Table 4, the base of the mountain is taken to be the Mare Imbrium floor, and in all other cases mountain bases are first determined coarsely from contours spaced 500m apart, and then fine-tuned with elevation masks. The base is the lowest elevation that enables the terrain encircling the mountain to remain 'independent' from surrounding features. As mentioned previously, that's only one way of doing it.All the peaks with asterisked names don't have official names. Which is surprising when we're talking about peaks that are so, well, prominent. 'Mons Elbruz' and 'Southern Farside Mountain' are names proposed by Danny Caes, and all the others are just nicknames I came up with - the rationale for them is in the detailed section for each peak further on.The fact that all these peaks belong to the rings of either the Imbrium or SPA basins is easily seen from their locations marked by stars in Figs 1 and 5 - they're distributed around the periphery of those basins.I've only listed mountains taller than Mons Huygens, often touted as the Moon's tallest peak. You'll come across a wide range of heights for it depending on source - from 4.6 km to 6.1 km, with 5.5 km seemingly the most common figure. That's quite a spread for a 5.3 km peak, and the misinformation around it has persisted for over a decade, despite evidence to the contrary available from LROC Quickmap. I look into this curious situation in the companion site The Mons Huygens Myth.It's also worth mentioning that besides the mountains listed in Table 4, there are a number of others around the SPA basin on the farside with base-to-peak heights between that of Mons Huygens, and Mons Mouton. Those shown in Table 4 are just a selection.
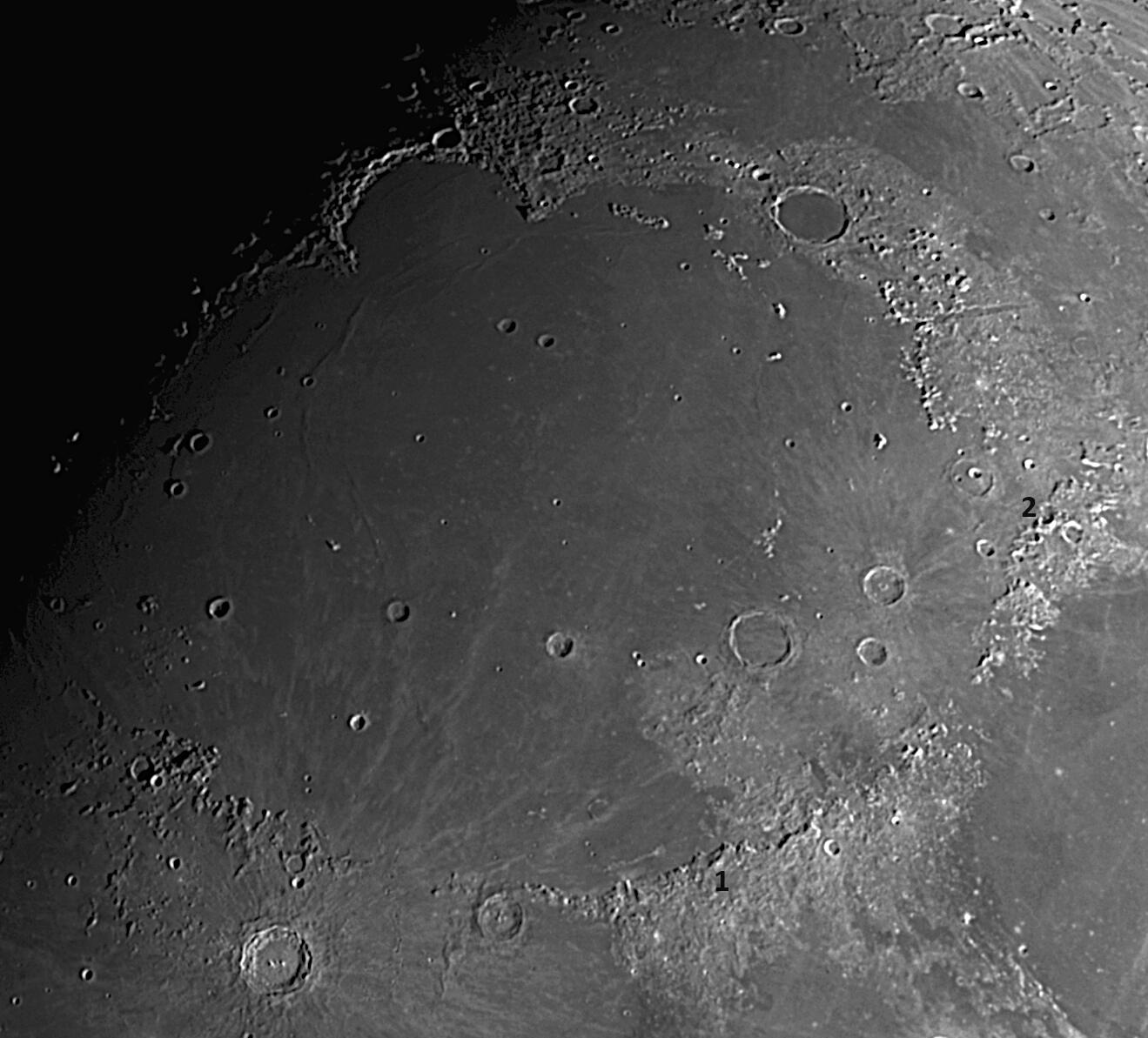
Fig 18: Mare Imbrium - telescopic image (8" untracked Dobsonian)
Fig 18 is an image I took of Mare Imbrium from my backyard with an 8" untracked Dobsonian telescope and basic astrocam. You can image both solar system objects (like the Moon, planets, comets) and deep-sky objects (such as galaxies, nebulae and star clusters) with this relatively simple and cost-effective combination - see here for details.The peaks '1' and 2' correspond to the ones marked the same on the nearside colour-coded lunar disk of Fig 1. The 'broken glass wall' section of the basin's rim in the lower right of the image is the celebrity Appenine range, and Mons Huygens is at the top tip of the '1'. The shorter Caucasus range along the centre right edge of the image is a lot less vivid but is home to a peak taller than Mons Huygens - Mons Elbruz - it's casting a semi-circular shadow on the mare, immediately to the right of the base of the '2'.Some key take-outs from Table 4 are:
-The tallest officially named mountain on the Moon is Mons Mouton. It's in the nearside south polar region.
-There are peaks even taller than Mons Mouton on the lunar farside, but they're as-yet unnamed.
-The peak elevations of the Moon's tallest mountains are modest compared with the elevations of its highest locations.
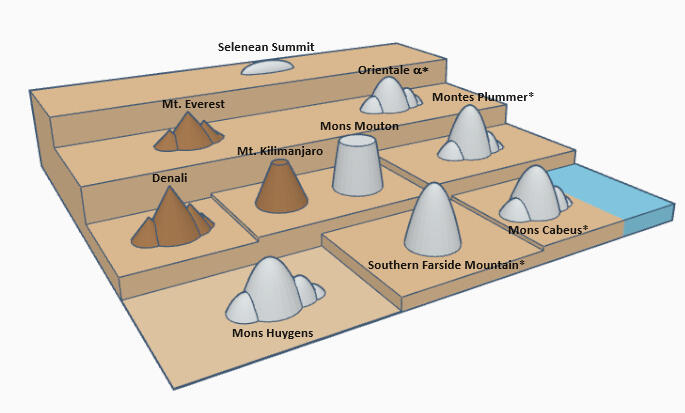
Fig 19: Mountains of the Earth and Moon - a comparison
Figure 19 depicts the relative elevations and base-to-peak heights of some of the highest and tallest lunar features discussed so far, with those of Earth.A mountain with a cluster of smaller peaks just denotes it's part of a range. Of the three free-standing peaks, Southern Farside Mountain has a 'classic' mountain shape, Kilimanjaro's a truncated cone on account of being a volcano, and Mons Mouton is a mesa-like formation (a raised table-topped structure).There's nothing on Earth at the height of the back row, and for this comparison there are no terrestrial features in the front row either, as that's terrain at or below sea level. Otherwise, the mountains of the two worlds aren't entirely dissimilar in elevation and base-to-peak height in the middle two rows. It's also clear that Southern Farside Mountain's base-to-peak height is greather than any mountain on the Moon or Earth, and all the lunar mountains in the first and second rows are taller than Mons Huygens. In the next section we'll take a closer look at each of the mountains in Table 4.
Mons Huygens
Mons Huygens is located on a ridge in the 550 km long arc-shaped Montes Appeninus (Appenines) range, which is part of the outer ring of the Imbrium basin. Fig 20 right panel shows its highest peak at 3.274 km above lunar datum at [Lat 19.526, Lon 357.096] to be on the rim of a 5 km crater, and it's also within 5 km of two other sub-peaks - one's 200m lower and the other 300m lower than the summit.The transect in Fig 20 left panel originates in the Appenine backslopes - an elevated area of ejecta from the Imbrium explosion. The transect cuts across Mons Huygens' summit, then down the Appenine front to the mare below. The mare is as flat as a pancake in this region of the Appenines, with an elevation almost dead on -2 km. The rubble of the backslopes also depletes the further back you travel from the Appenine front, until you're down to mare level again, as can be seen in Fig 18. No matter which way you go from the summit of Mons Huygens, you have to descend to the mare if you want to climb a higher peak. So per the height profile in Fig 21 along the transect, the base-to-peak height of Mons Huygens is 2.025+3.274 = 5.3 km.Fig 22 is an oblique 3D view of Mons Huygens from the mare. It corresponds to the transected region in Fig 20 left panel. The summit is marked with a blue circle.The Appenine front isn't smoothly continuous like 'conventional' crater rims. It may have been like that originally, but is serrated and irregular now as a result of collapsed blocks and landslides from the peaks.
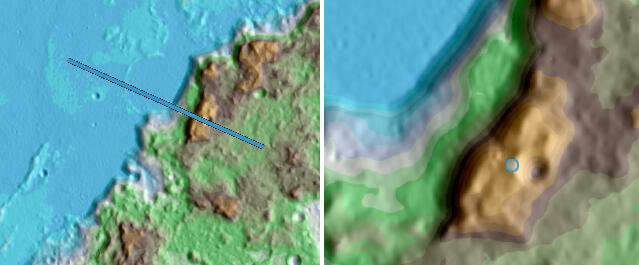
Fig 20: Mons Huygens - colour-coded terrain view (LROC Quickmap)
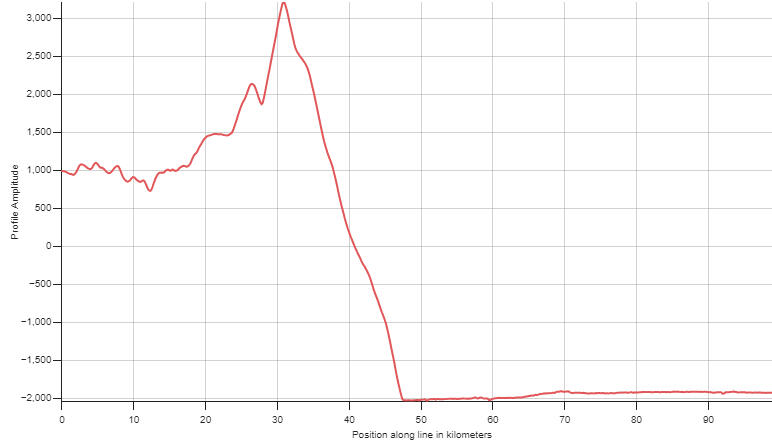
Fig 21: Mons Huygens - height profile (LROC Quickmap)
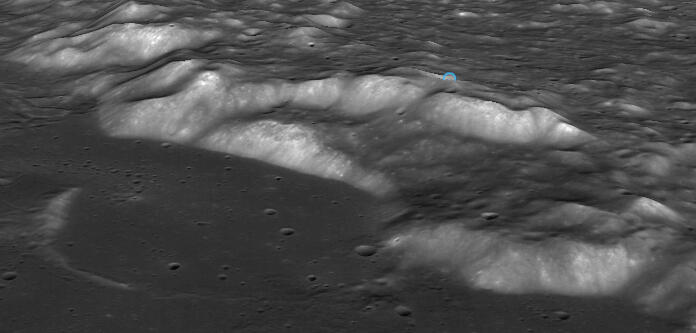
Fig 22: Mons Huygens - 3D terrain view (LROC Quickmap)
Drygalski Calipers*
I've called this ridge 'Drygalski calipers' not just because it looks like calipers and it's next to the farside crater Drygalski, but also that the 'calipers' appear to actually be measuring the crater - its 'jaws' are 120 km apart, which is the diameter of Drygalski's floor.The lowest contour that fully encircles this feature is 0 km, as highlighted by the black dots in Fig 23. However, Figure 25 shows that the formation remains distinct when terrain at -200m and higher is displayed, with anything lower blanked out. So, -200m can be considered the base.The transect in Fig 23 cuts across the higher of the two 'jaws' - its summit has an elevation of 5.526 km at [Lat -76.929, Lon 260.074]. Fig 24 shows the height profile along the transect, which traces out a mountain with a base-to-peak height of 0.2+5.526 = 5.73 km.Fig 26 is an oblique 3D view of this mountain - the transect across it corresponds to that in Fig 23.
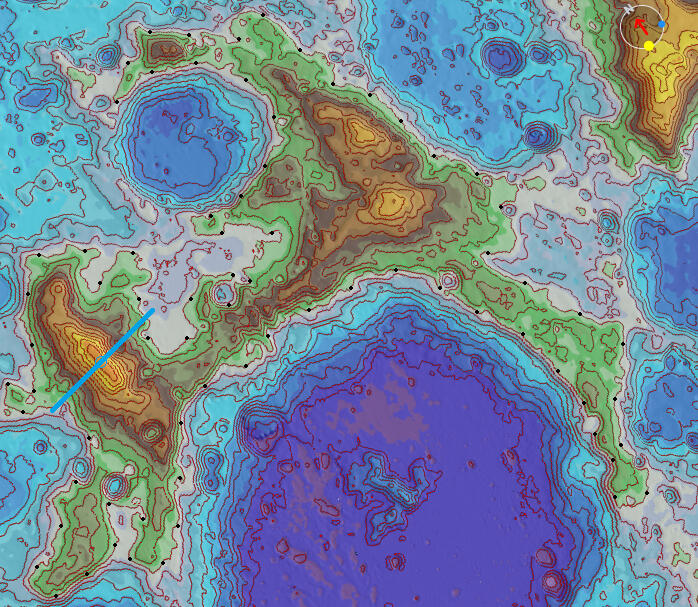
Fig 23: Drygalski Calipers* - elevation contour (LROC Quickmap)
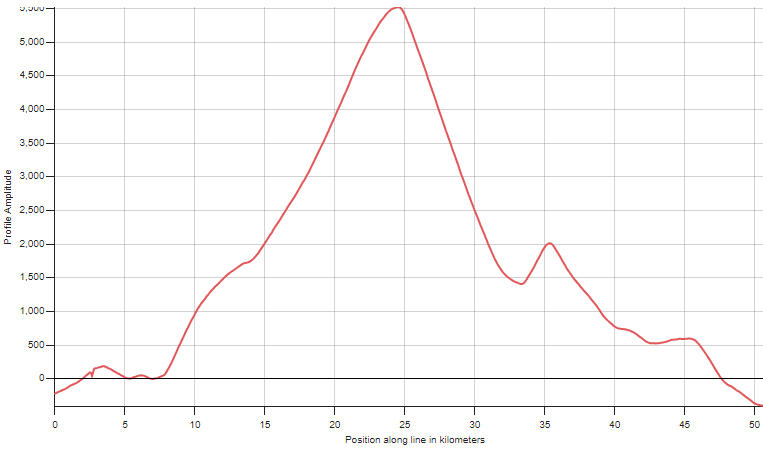
Fig 24: Drygalski Calipers* - height profile (LROC Quickmap)
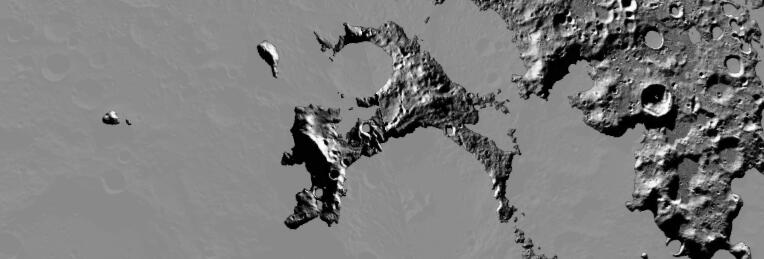
Fig 25: Drygalski Calipers* from base (LROC Quickmap)
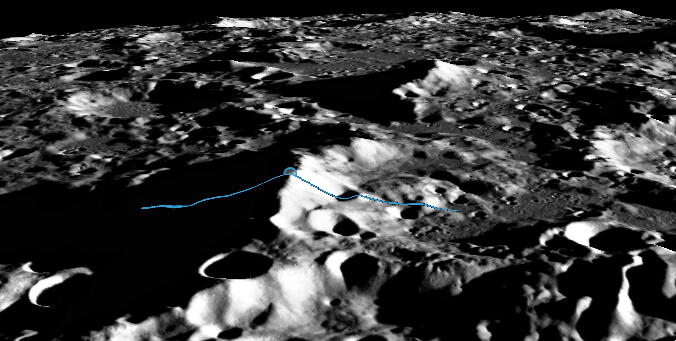
Fig 26: Drygalski Calipers* - 3D terrain view (LROC Quickmap)
Mons Elbruz*
The Caucasus mountains are likely part of the Imbrium basin's outer ring, as suggested by the fact they are sharpest and highest along their western edge, facing Imbrium. However, as clearly seen in Fig 18, they've somehow rotated a few degrees to the east - they don't follow the circle outlined by the Appenines to their south, and the Alps to their north. Also although the Caucasus aren't as rugged as the Appenines, there's a couple of craters in their midst with particularly high rim segments: 33 km Calippus has a ridge higher than 2 km along its north to east circumference. To the west of Calippus is a partial crater of similar size facing Imbrium - Calippus C. Its east half is missing but its western rim is flanked by another curved ridge as long as the one around Calippus. But at an elevation of 3.625 km, this ridge's highest peak bests Calippus' by 1 km. Calippus C's east side may have got uplifted with the Caucasus, but its western half covered by the later lava flows which filled Imbrium.The mare floor at the Caucasus front is more rubbly than in Mons Huygens' vicinity, but per the height profile in Fig 28, the roughness still only shows up as small fluctuations around a -2 km elevation. Which makes the base-to-peak height of the Calippus C ridge: 2.018+3.625 = 5.64 km.The peak has been designated Caucasus Alpha (α) in old Moon maps, but I like Danny Caes' proposed name: Mons Elbruz. Not just because Mount Elbrus is the highest peak of Earth's Caucasus mountains (which the lunar ones are named after), but its elevation of 5.64 km is exactly the same as Mons Elbruz' prominence!
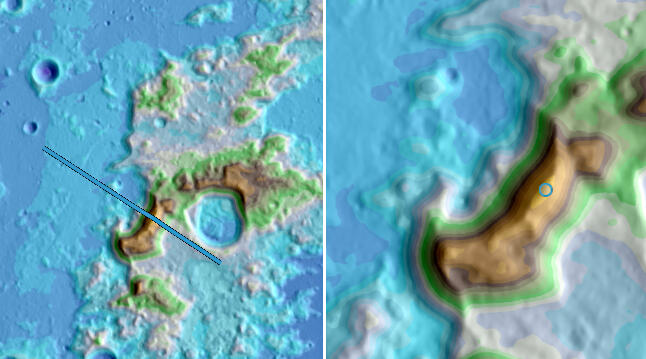
Fig 27: Mons Elbruz* - colour-coded terrain view (LROC Quickmap)
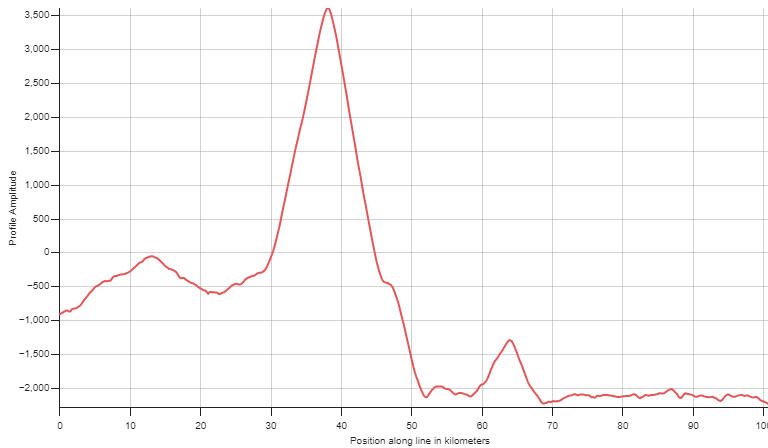
Fig 28: Mons Elbruz* - height profile (LROC Quickmap)
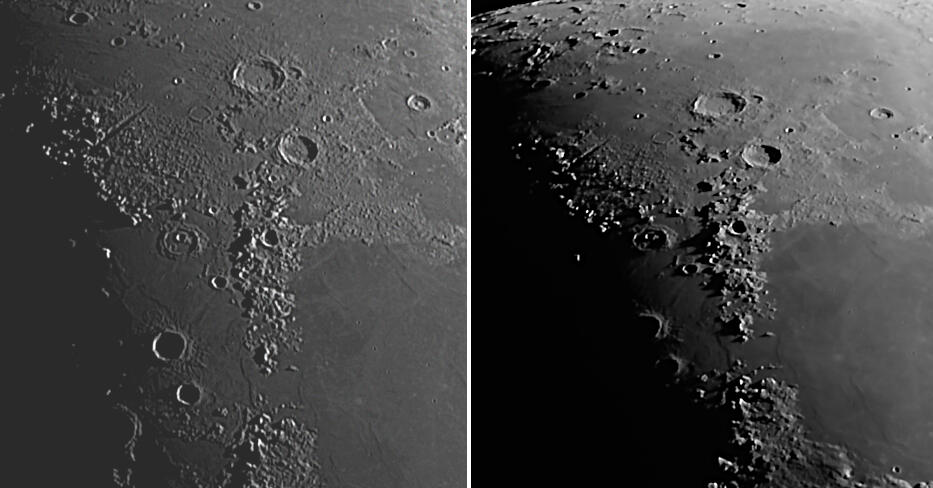
Fig 29: Mons Elbruz* region - telescopic images (8" untracked Dobsonian)
The best time for telescopically observing or imaging lunar mountains is when the terminator, the boundary between lunar night and day, is nearby. This maximizes the contrast, creating a striking 3D effect that emphasizes the mountains. To illustrate, Fig 29 presents a couple of images I took from my backyard - Monz Elbruz is in the centre of both, but the sun is a little lower in the image on the right and so the Caucasus 'pop out' more. But this is also the time mountains cast long, distinct shadows - note Mons Elbruz' shadow stretching some 90 km to the west towards Cassini crater. This can create a misleading impression of jagged lunar mountains, as depicted for example by James Nasmyth in the nineteenth century in Fig 30 left panel, and at by Chesley Bonestell at the dawn of the space age in Fig 30 right panel.
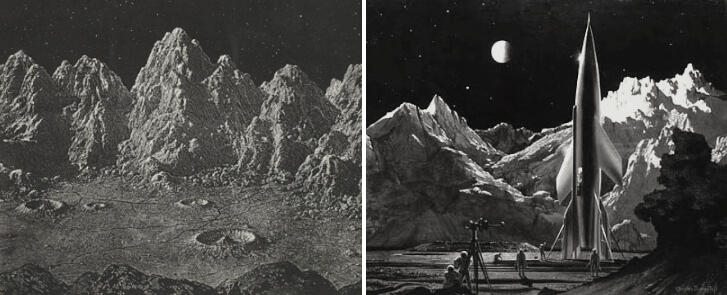
Fig 30: Imagined Moon mountains
Fig 30 left: 'Group of Lunar Mountains' - James Nasmyth (1874)
Fig 30 right: 'Conquest of Space' - Chesley Bonestell (1949)
When lunar mountains first formed billions of years ago they may have been as severe-looking as what the pictures in Fig 30 suggest, but now slopes rarely exceed 30°. The oblique 3D view of Mons Elbruz in Fig 31 is pretty representative of what lunar mountains are really like. Mons Elbruz slopes 22° from its Mare Imbrium base to summit, and Mons Huygens 18°. The much gentler profile is a result of several factors: for a start there's no wind or water on the Moon to carve sharp peaks and cliffs. In addition, constant sandblasting from micro-meteorite impacts, weathering from solar wind and cosmic rays, seismic shaking and fracturing of rock from extreme temperature swings gradually smooth out lunar features over time, further contributing to their rounded appearance.
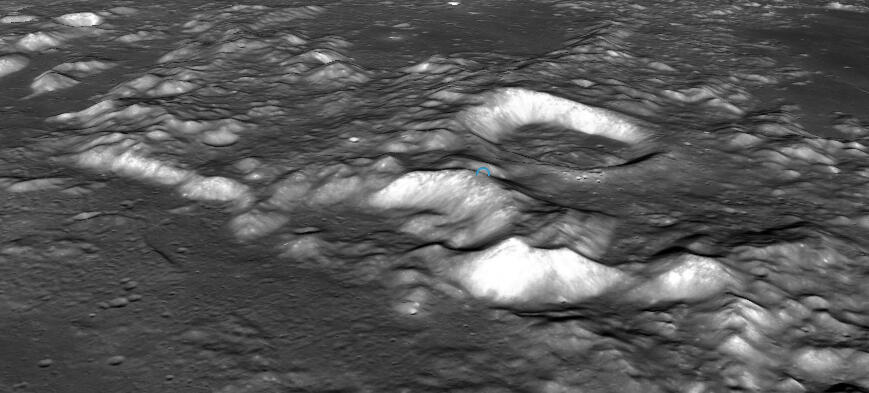
Fig 31: Mons Elbruz* - 3D terrain view (LROC Quickmap)
Mons Cabeus
This ridge is the first of two entries in Table 4 located in the nearside's south polar region. It's perched on the northern rim of Cabeus crater, so I've simply called it Mons Cabeus.
(edit: this peak got officially named Mons Cabeus by the IAU in March 2025)The lowest contour that fully encircles this feature is 0 km, as highlighted by the black dots in Fig 32. However, Figure 34 shows that the feature remains independent when terrain at -150m and higher is displayed. So, -150m can be considered the base.Mons Cabeus' top features two peaks 6.5 km apart. Per the height profile in Fig 33 along the transect in Fig 32, the summit at [Lat -83.608, Lon 325.971] is 120m higher than the adjacent peak and 5.689 km above the base. So we have a base-to-peak height of 0.15+5.689 = 5.84 km.See Fig 42 for an oblique 3D view of the mountain.
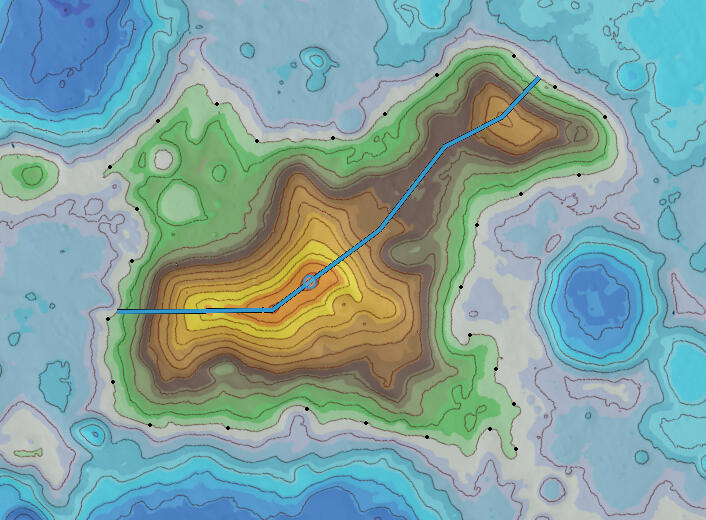
Fig 32: Mons Cabeus - elevation contour (LROC Quickmap)
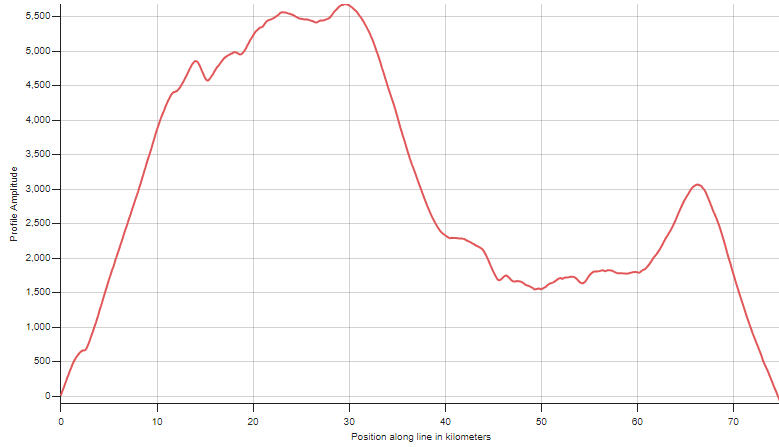
Fig 33: Mons Cabeus - height profile (LROC Quickmap)
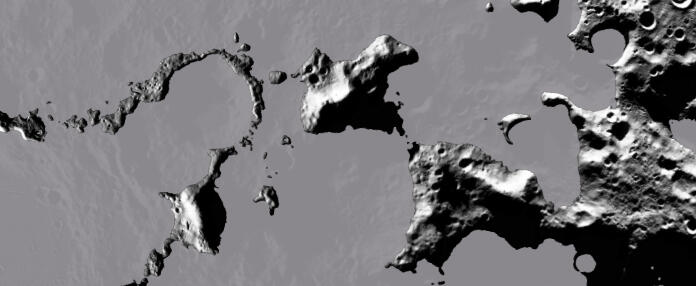
Fig 34: Mons Cabeus from base (LROC Quickmap)
Mons Twin Peaks*
Of all the ridges that could be called Twin Peaks, I felt this one to be the most deserving. The two peaks in this farside formation are very distinctive - they rise more than 2 km above their common col, are quite well separated at 25 km apart, and are both taller than Mons Huygens from the base (the shorter one just).As highlighted by the black dots in Fig 35, a -1 km contour fully encircles the formation. Fig 37 also confirms that the feature just remains independent when terrain at elevation -1 km and higher is displayed. So, -1 km can be considered the base. The summit at [Lat -48.195, Lon 227.468] is 4.785 km above this base, giving a base-to-peak height of 1+4.785 = 5.78 km.
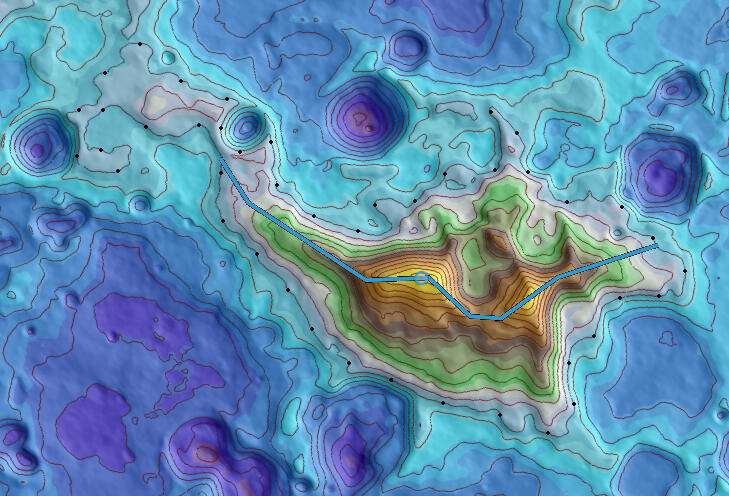
Fig 35: Mons Twin Peaks* - elevation contour (LROC Quickmap)
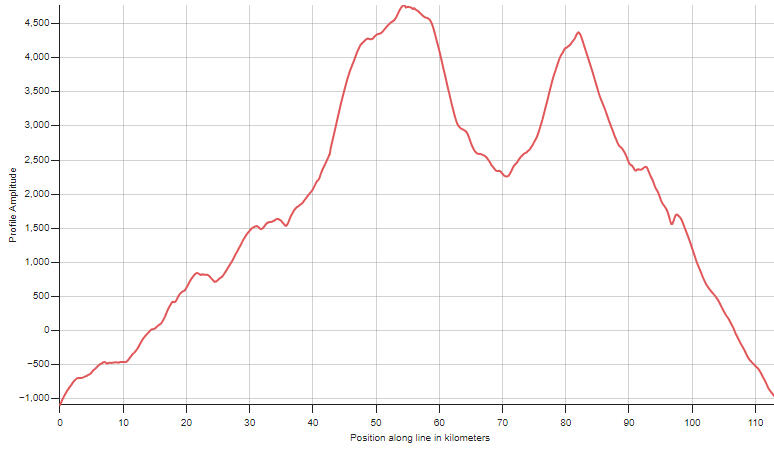
Fig 36: Mons Twin Peaks - height profile (LROC Quickmap)
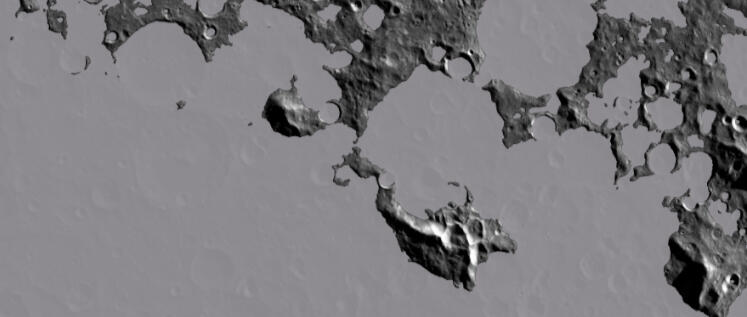
Fig 37: Mons Twin Peaks* from base (LROC Quickmap)
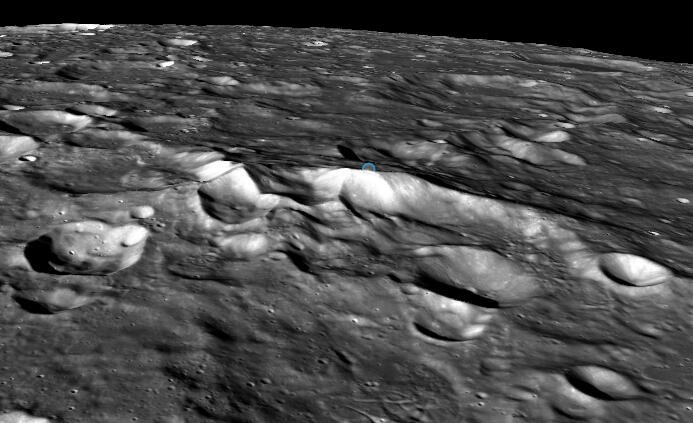
Fig 38: Mons Twin Peaks* - 3D terrain view (LROC Quickmap)
Mons Mouton
Formerly informally called Leibnitz Beta (β), Mons Mouton is the second of two entries located in the nearside's south polar region. As can be seen in Figs 39-42, its top is a plateau, so its 7.026 km summit at [Lat -84.673 , Lon 39.475] is almost imperceptibly higher than what's around it.As highlighted by the black dots in Fig 39, a 1 km contour fully encircles the mountain. Fig 41 also confirms that this feature just remains independent when terrain at elevation 1 km and higher is displayed. So, 1 km can be considered its base.Which makes Mons Mouton 7.026-1 = 6.03 km tall. Fig 42 presents an oblique 3D view of it.
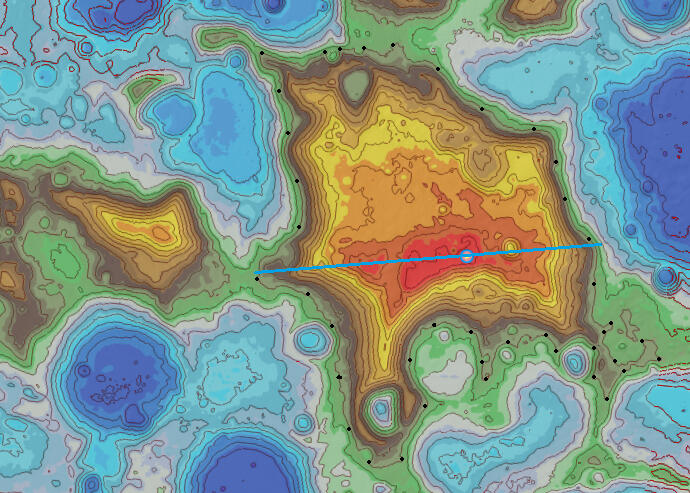
Fig 39: Mons Mouton - elevation contour (LROC Quickmap)
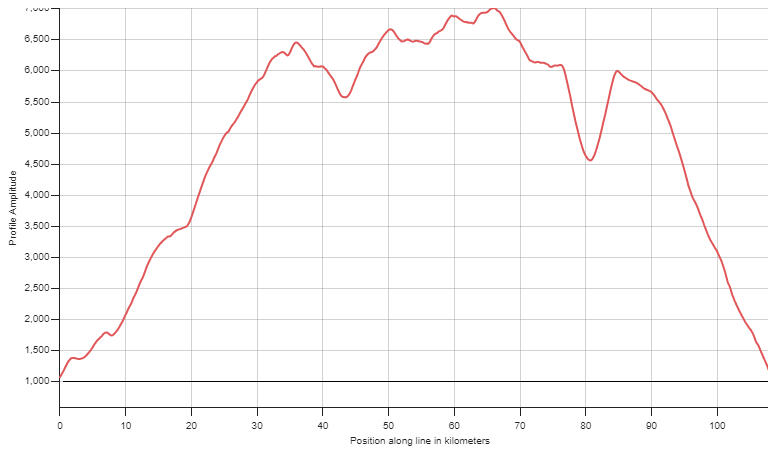
Fig 40: Mons Mouton - height profile (LROC Quickmap)
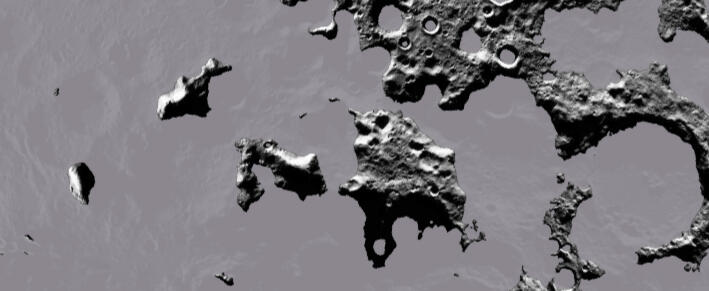
Fig 41: Mons Mouton from base (LROC Quickmap)
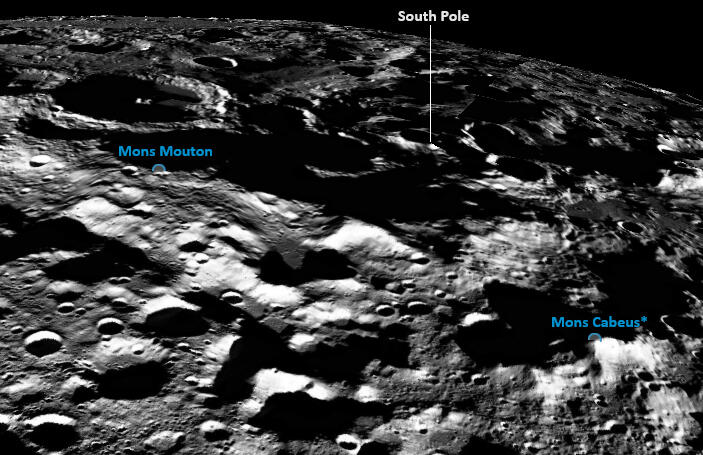
Fig 42: South Polar region - 3D terrain view (LROC Quickmap)
Montes Plummer*
I've named this farside mountain chain for the largest and freshest impact to have punched through it - the 77 km complex crater Plummer, seen along the bottom right edge of Fig 43 and right edge of Fig 46. It's an irregular formation of connecting ridges with many sub-peaks, which also happens to be located in the highest terrain.The dotted contour which fully encircles it in Fig 43 is at an elevation of 2 km. However, Fig 45 shows that the feature remains an 'island' when terrain at elevation 1.65 km and higher is displayed. So, 1.65 km can be considered the base of this system.Montes Plummer's summit at [Lat -22.726 , Lon 204.132] is 8.564 km from the lunar datum, so its base-to-peak height is 8.564 - 1.65 = 6.91 km. No higher peak on the Moon is this tall.
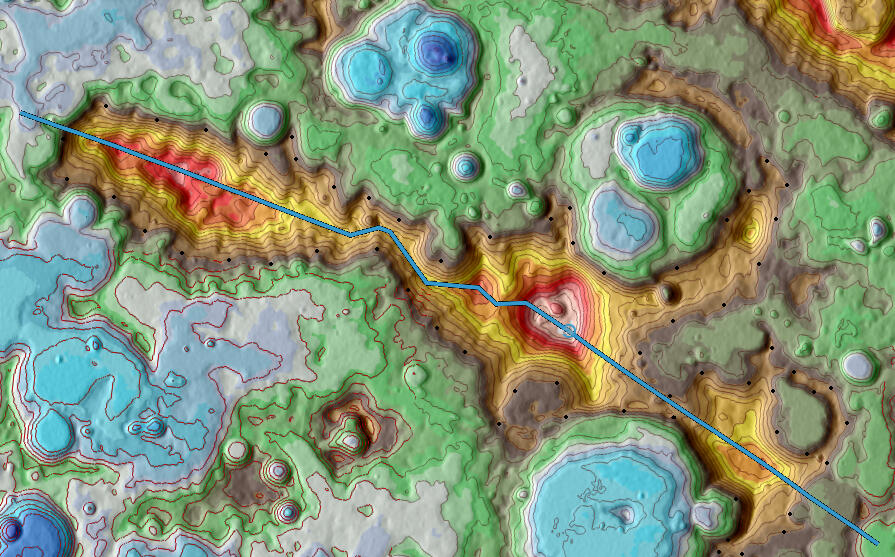
Fig 43: Montes Plummer* - elevation contour (LROC Quickmap)
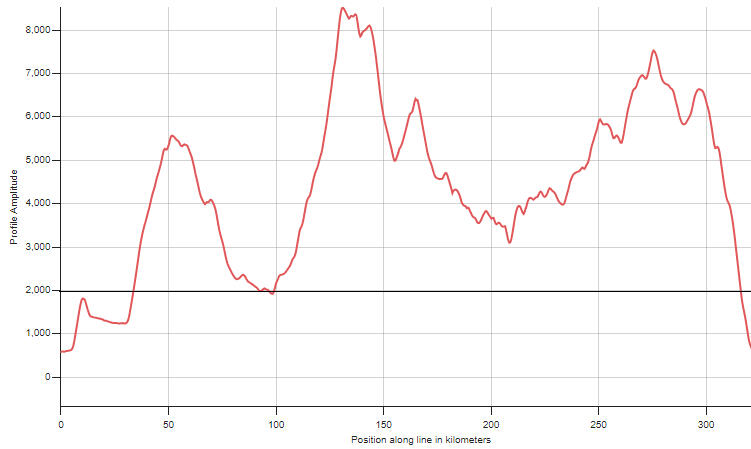
Fig 44: Montes Plummer* - height profile (LROC Quickmap)
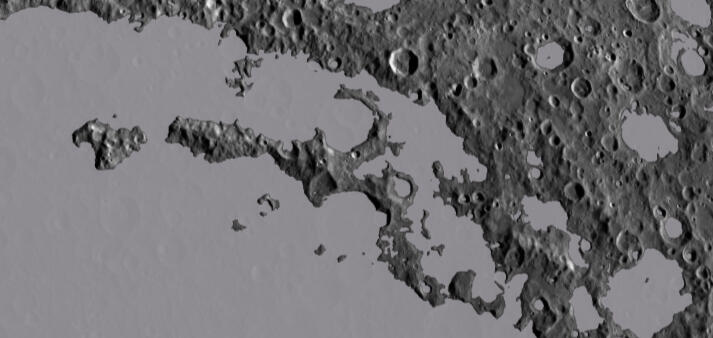
Fig 45: Montes Plummer* from base (LROC Quickmap)
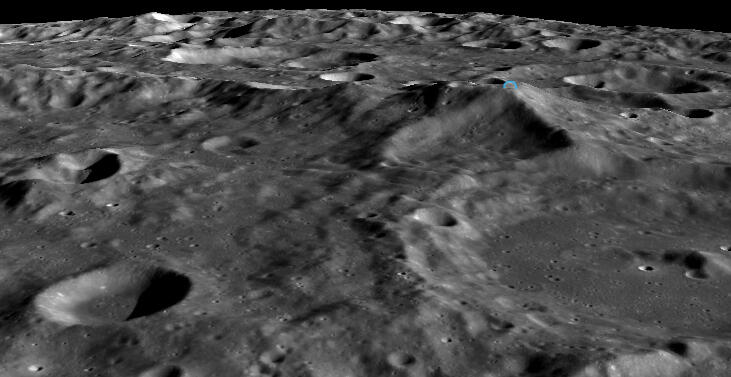
Fig 46: Montes Plummer* - 3D terrain view (LROC Quickmap)
It's speculated that the SPA basin may have had as many as four rings when it formed, and of the entries in Table 4, Montes Plummer is the longest continuous part of one of them. For its size, the basin should actually be deeper than it is, and its peaks even taller. One explanation might be that the projectile that formed the basin was slower moving than normal and hit at a shallow angle. There are other signs that support this theory, like the basin having an oval outline rather than a circular one, and less impact melt and excavated mantle material in its interior than expected. So had this been a full-on hypervelocity impact, we could now be looking at a more striking basin, more pronounced multi-ring pattern, and more significant peaks. It's even been suggested that such an impact could have come close to breaking the Moon apart!But even as is, it's staggering to consider the 17.69 km difference in elevation within the SPA basin, from the highest point on its rim - Montes Plummer, which on Earth would be less than 300m below Mount Everest's summit - to its lowest point, Point Trieste.
Southern Farside Mountain*
I've gone with the name Danny Caes has suggested for this farside icon. There's perhaps a case for making the name a little more specific - as we've seen, there's more than one tall mountain in the southern farside.Per Fig 47, the dotted 0 km contour neatly encircles this mountain. However, Fig 49 shows that the feature remains detached when terrain at elevation -400m and higher is displayed. So, -400m can be considered its base.The mountain's summit at [Lat -50.126, Lon 236.785] has an elevation of 6.739 km. So, its base-to-peak height is 0.4+6.739 = 7.14 km.
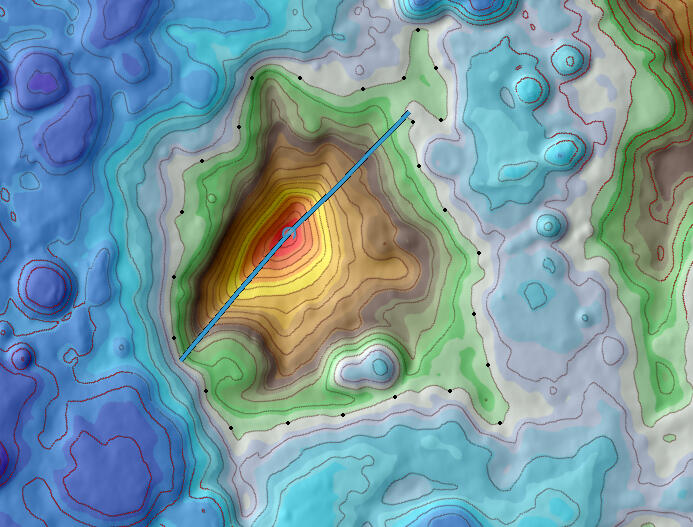
Fig 47: Southern Farside Mountain* - elevation contour (LROC Quickmap)
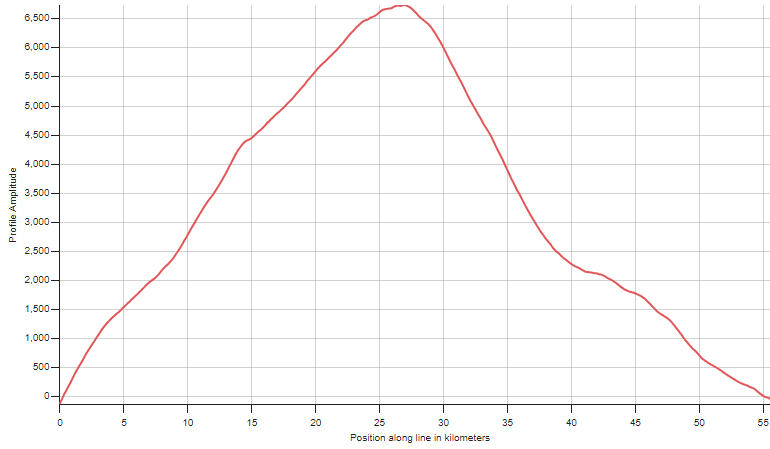
Fig 48: Southern Farside Mountain* - height profile (LROC Quickmap)
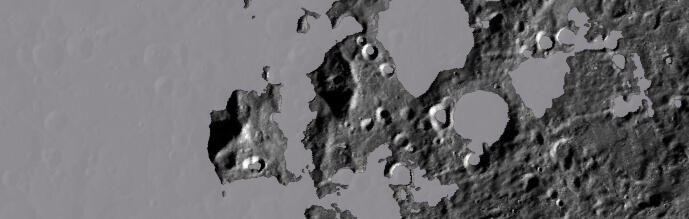
Fig 49: Southern Farside Mountain* from base (LROC Quickmap)
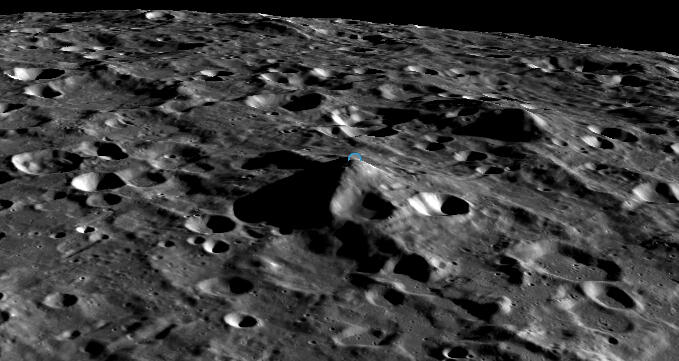
Fig 50: Southern Farside Mountain* - 3D terrain view (LROC Quickmap)
This mountain seems the very definition of one, so it's intriguing that it remains unnamed by the IAU:...a landform that rises prominently above its surroundings: Check. It's the tallest free-standing mountain on the Moon.
...generally exhibits steep slopes: Check. Its north-western slope is about as steep as Mons Huygens'.
...has a relatively confined summit area: Check. It rises steadily from all sides to a well-defined apex.For comparison, Fig 51 is the height profile of the tallest free-standing mountain on Earth - Mount Kilimanjaro. It's 5.9 km high, rising from a plateau about 1 km high, making the base-to-peak height a landscape-commanding 4.9 km. See also Fig 19 for a simulation of how it measures up against other mountains. Can you imagine this wonder not being named?
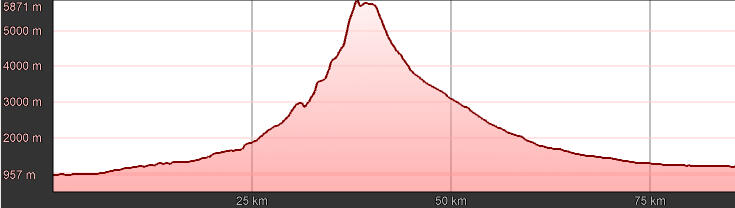
Fig 51: Mount Kilimanjaro - height profile (Google Earth Pro)
Even among peaks within ranges, very few stand out more from their surrounding terrain than Kilimanjaro. Mount Everest's base-to-peak height for example is on average 3.8 km, if its base is considered the Tibetan plateau, as Figs 19 and 56 show. Denali in Alaska, however, is one peak that surpasses Kilimanjaro in this measure. With a summit reaching 6.19 km above sea level and a sloping plains base with an average elevation of about 700m, Denali stands roughly 5.5 km above its base. So Denali, arguably the tallest mountain on Earth's dry land, would just manage to scrape into our list of tallest lunar peaks in Table 4.The tallest free-standing mountains on Earth, like Kilimanjaro, tend to be strato-volcanoes. These don't exist on the Moon because they're a product of colliding tectonic plates, and the Moon is a one-plate body. Kilimanjaro's upward-steepening (concave) slope is typical of strato-volcanoes and results from factors like earlier lava flows being more extensive than later ones, and progressive erosion of material from the volcano's flanks towards its base, intensified by eruptive events and earthquakes.While the Moon does have elevated volcanic features known as domes, they're a lot smaller than Earth's strato-volcanoes. Fig 52 shows a few different types that I imaged from my backyard. The ones depicted in the top left panel are typical - they're low, wide, hemispherical structures with diameters ranging from 5 km to 15 km, heights in the hundreds of meters, and very gentle slopes up to 5°. Some have summit pits. They're composed of runny lava, which spreads thinly and doesn't accumulate easily.Contrasting with the classic domes, examples like the Arago Beta (β) dome in the Sea of Tranquility (top right panel) are larger, steeper, rougher and irregular. Their shape could be a result of small lava volumes oozing to the surface through multiple fractures, and their size and texture could be down to variations in the type and temperature of lava in the region.The Rumker Hills (bottom left panel), a cluster of domes atop a gently elevated mound, may owe their form to subsurface magma uplifting the area, or lava piling up around surface vents, or a combination of both.The Gruithuisen domes (bottom right panel) stand out, literally, as the Moon's tallest volcanic formations. Unlike typical mare lava, which is basaltic and no thicker than motor oil, these domes are composed of silica-rich lava that is more viscous. And because it's thicker, it moves slower and cools faster, and so is able to pile up into structures this size.
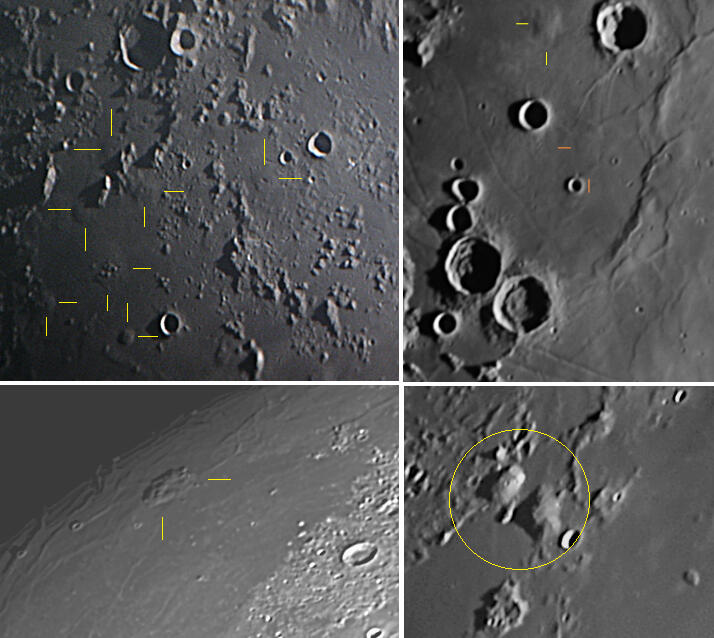
Fig 52: The Moon's volcanic domes - telescopic images (8" untracked Dobsonian)
Fig 52 Top left: 'Domeland' around Milichius crater, west of Copernicus. The domes peak at 100-230m above mare level
Fig 52 Top right: Arago Beta (β) dome. It's 300m high, 20km diameter. Just south of it, an unnamed dome is highlighted with orange markers. It's of similar diameter to Arago Beta, but nearly 100m shorter
Fig 52 Bottom left: Mons Rumker. Its peak is 1.3 km above mare level, 70 km diameter
Fig 52 Bottom right: Gruithuisen domes are circled. In the centre of the image is Delta (δ), to its north-west is Gamma (γ), and the smaller dome to Gamma's north throwing the triangular shadow is the 'North-West' dome. Delta rises 1.9 km above the mare, 30 km diameter. Gamma is a little shorter than Delta - its peak is 1.75 km above mare level, 23 km diameter. The 2 km crater on Gamma's summit is not a volcanic vent, but an impact crater that just happens to be centrally placed
But none of these domes rival the loftiness of the Moon's tallest mountains. So if those mountains aren't volcanic, why do some of them present as short ridges or even solitary peaks? It's because the rings of impact basins like Imbrium and SPA, where these peaks lie, are not as complete or well-defined as those of newer basins. For example Oriantale in Fig 14, being one of the Moon's most recent basins, remains well-preserved, but its peaks are comparatively smaller because the basin is. The size of a crater corresponds to its age; thus, the youngest basin, Oriantale, is the smallest, while the oldest, SPA, is the largest. Over time, impacts become less frequent and occur with smaller projectiles. So the older and larger basins are more likely to be degraded from subsequent large impacts, which can obliterate or obscure large portions of the rings, such as around SPA, or from mare volcanism, which can flood the interior depression with lava, reducing the extent of the rings or even burying parts of them, as seen in Imbrium.Speaking of mare volcanism, the most common kind of volcano on the Moon actually looks the least like one - a 'flood basalt' volcano - basically a stretch of land covered by widely spread lava from a single or series of eruptions originating from one or more fractures. Even thin lava, if it flows fast enough and for long enough, can create pretty thick slabs - like up to a few kilometers thick in Imbrium. As a result, the base of Mons Huygens for example is now at mare level, higher than when the basin first formed, and so Mons Huygens isn't as tall as it once was. Why a third of the nearside experienced flood basalt volcanism but the farside didn't, remains a puzzle in lunar science.
This is probably an opportune time to touch on an interesting case - the informally named Zeeman Mons - star '8' in Fig 5.
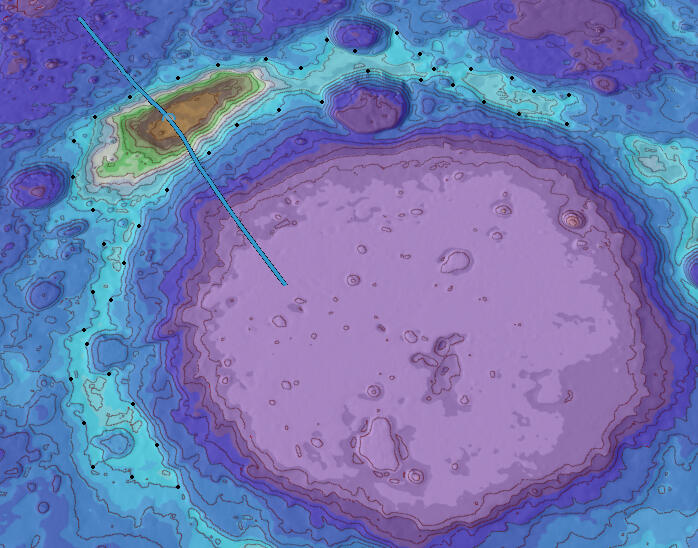
Fig 53: Zeeman Mons* - elevation contour (LROC Quickmap)
Zeeman Mons is situated on the rim of the 185 km farside crater Zeeman, and as outlined by the black dots in Fig 52, a -2 km contour fully encircles the formation. You could walk from Zeeman Mons' summit to the highlands proper without descending much more than -2 km. Its summit at [Lat -72.962, Lon 215.029] has an elevation of 2.487 km, making Zeeman Mons' base-to-peak height 2+2.487 = 4.49 km. Although that doesn't qualify Zeeman Mons for inclusion in our list of the Moon's tallest peaks in Table 4, it's still a decent mountain that really 'sticks out' from the rest of Zeeman crater's rim. So it may not have resulted from the Zeeman impact. It might be a remnant of one of the SPA basin's rings.The other thing about Zeeman Mons is that there's a significant change in terrain elevation on either side of it. As the profile graph in Fig 54 shows, the landscape descends to -4 kilometers approximately 40 km northwest of the summit and plunges to just over -6.3 km some 60 km southeast of it. So one rock face ascends 4+2.487 = 6.49 km from the SPA basin floor, while another rises 6.32+2.487 = 8.8 km from Zeeman's floor.
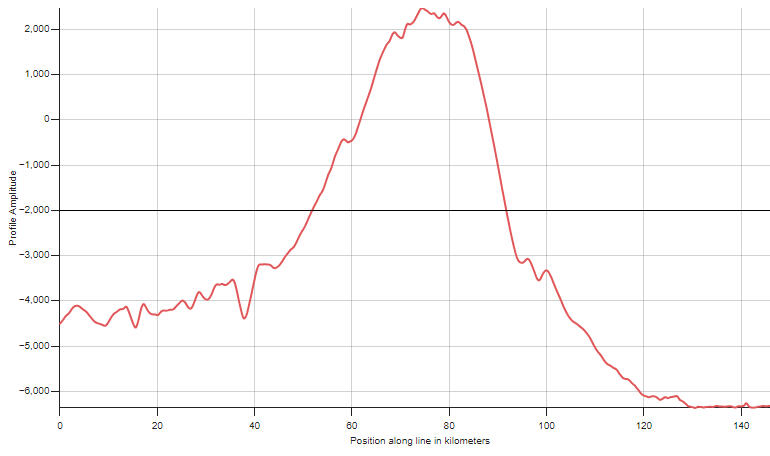
Fig 54: Zeeman Mons* - height profile (LROC Quickmap)
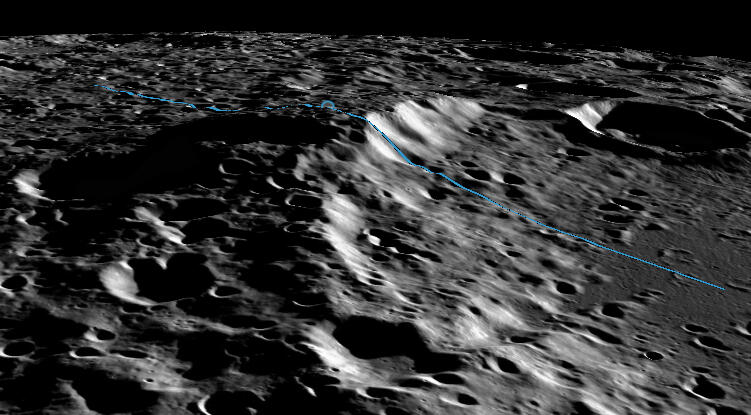
Fig 55: Zeeman Mons* - 3D terrain view (LROC Quickmap)
Now, 8.8 km also happens to be approximately Mount Everest's elevation. Unlike Zeeman Mons though, where an 8.8 km drop occurs within a 60 km stretch, descending from Everest's summit to sea level takes 690 km, as the nearest sea is the Bay of Bengal. The final descent of just 100 meters to sea level covers nearly half of that 690 km distance, but we can descend most of Everest's height in a much shorter distance than that. Consider Fig 56 - the height profile from Everest's peak to the Arun river valley in Nepal. It displays an elevation change of 8.5 km over 75 km. That's probably the greatest height difference between any two points this close together above sea level on Earth.
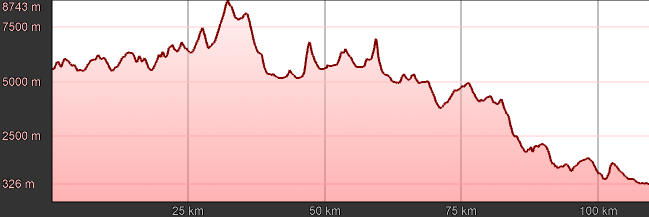
Fig 56: Mount Everest to Arun river valley - height profile (Google Earth Pro)
Fig 56 prompts the following comparative questions with lunar topography:Q1. A change in elevation of 8.5 km can be experienced over a horizontal distance of 75 km on Earth. What is the greatest change in elevation on the Moon over a similar distance?A. In the nearside south polar region, it's a 10.9 km drop from the peak of Mons Mouton to the floor of adjacent Scott crater, spanning 77 km:
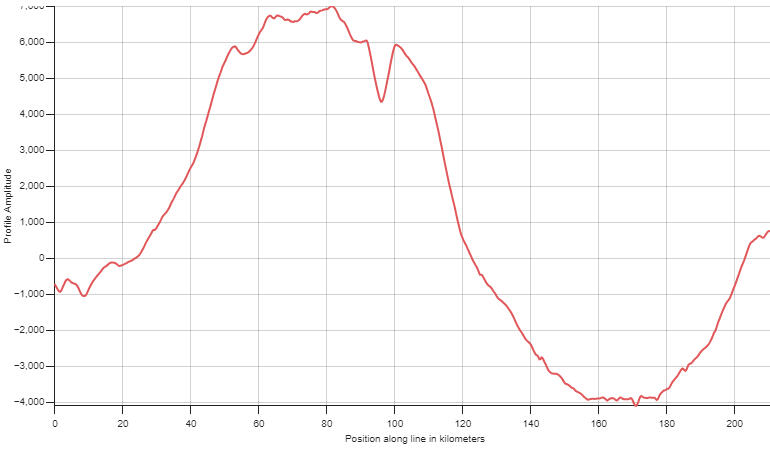
Fig 57: Mons Mouton and Scott crater - height profile (LROC Quickmap)
But on the farside, we can better that. From the summit of Southern Farside Mountain to the nearest deep crater, it's a whopping 6.739+5.258 = 12 km change in height in just 68 km. The crater in question is the 15 km fresh-rimmed one you can see in Fig 50, close to the mid left edge of the image.
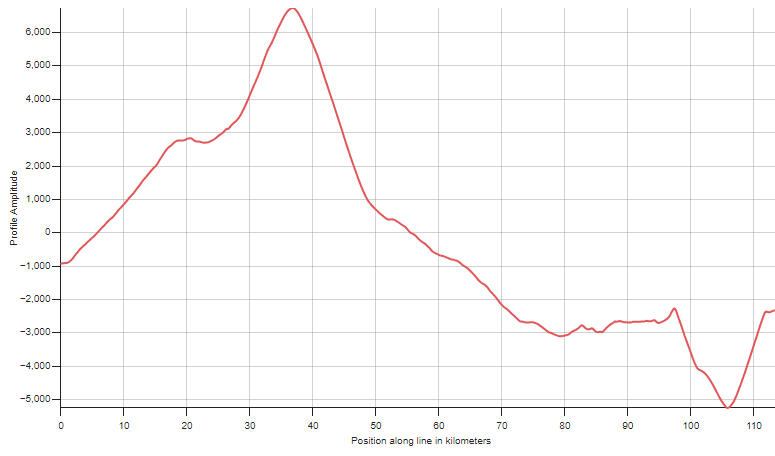
Fig 58: Southern Farside Mountain* and unnamed crater - height profile (LROC Quickmap)
So this is like descending from Aconcagua, the highest elevation outside Asia, to the average depth of the Pacific Ocean, within the equivalent horizontal distance of crossing from one side of a city like Dallas, Texas, to the other!
Q2. A change in elevation of 8.5 km can be experienced over a horizontal distance of 75 km on Earth. What is the shortest distance over which at least this change in elevation occurs on the Moon?A. Consider Aitken - star '9' in Fig 5. This 135 km complex crater already has a 'high profile' for being the 'A' in SPA basin, and a focus of NASA's Artemis program for having features that are unusual for the farside (like a lava filled floor, irregular mare patches and wrinkle ridges). But its Aitken's high profile in Fig 60 that's of particular interest here.
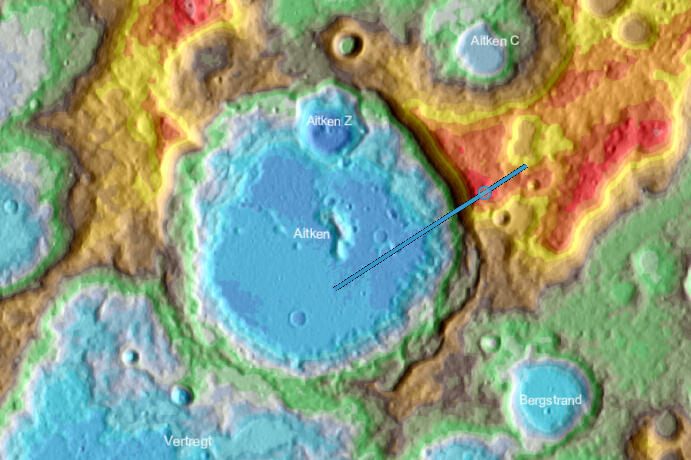
Fig 59: Aitken crater - colour-coded terrain view (LROC Quickmap)
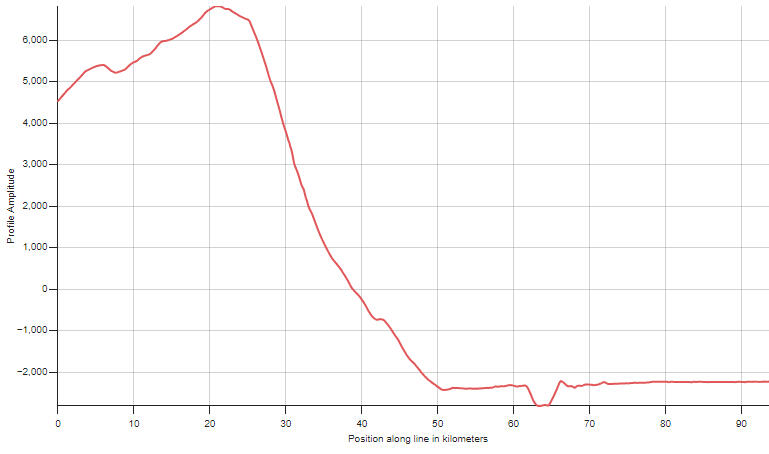
Fig 60: Aitken crater - height profile (LROC Quickmap)
The profile shows a change in elevation of 9.26 km in just 30 km, along the transect in Fig 59!The transect passes through the highest point on Aitken's eastern rim at [Lat -15.897, Lon 175.394] with an elevation of 6.385 km, touches Aitken's floor at an elevation of -2.429 km, and finishes a couple of hundred meters higher just south of the central peak. While Zeeman which we looked at earlier features a lower rim ridge but deeper floor than Aitken, Aitken's elevation change surpasses it over a shorter distance.So this part of Aitken's wall slopes almost as steeply as Mons Huygens, but rises 4 km higher in sheer rock face.For a sense of scale, in Fig 61 the silhouette of the Empire State Building on Aitken's floor is dwarfed by the 1.8 km high central peak next to it, to say nothing of the colossal 9.26 km high crater wall 65 km in the background.
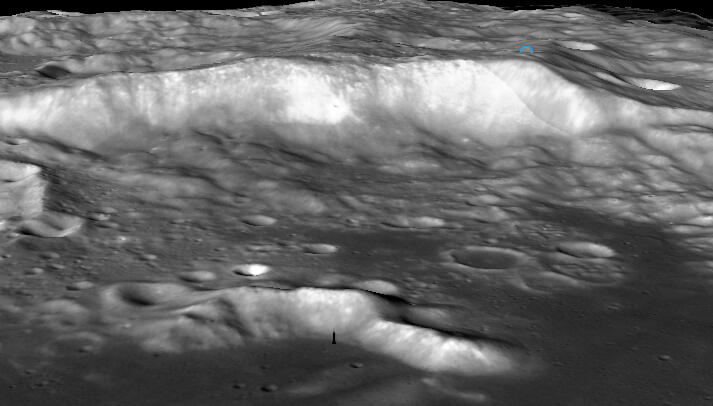
Fig 61: Aitken crater - 3D terrain view (LROC Quickmap)